Figure 1: Schematic description of the correlation between electrical conductivity and gallium excess (a), geometrical structure (b), and band structure (c) of highly non-stoichiometric and amorphous gallium oxide as a function of temperature. As the temperature increases, β-Ga2O3 nuclei start to crystallize at Tcryst and both the gallium excess and the related Fermi energy, EF, in the amorphous oxide increase. Once the gallium excess reaches its critical value, the bandgap is closed and the insulator-metal transition occurs at the temperature TIM.
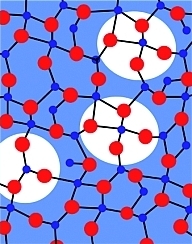
Lakshmi Nagarajan1, Roger A. De Souza1, Dominik Samuelis1,5, Ilia Valov2, Alexander Börger3, Jürgen Janek2, Klaus-Dieter Becker3, Peter C. Schmidt4 and Manfred Martin1
1 Institute of Physical Chemistry, RWTH Aachen University, 52056 Aachen, Germany
2 Institute of Physical Chemistry, Justus Liebig University, 35392 Giessen, Germany
3 Institute of Physical and Theoretical Chemistry, Technical University of Braunschweig, 38106 Braunschweig, Gerrmany
4 Institute of Physical Chemistry, Technical University of Darmstadt, 64287 Darmstadt, Germany
5 Present address: Max Planck Institute for Solid State Research, Heisenbergstr. 1, 70569 Stuttgart, Germany
Published as: “A chemically driven insulator–metal transition in non-stoichiometric and amorphous gallium oxide”, Nature Materials Vol. 7, 391-398 (2008)
Insulator–metal transitions are well known in transition metal oxides, but inducing an insulator–metal transition in the oxide of a main group element is a major challenge. We succeeded in demonstrating that highly nonstoichiometric, amorphous gallium oxide shows an insulator–metal transition, with a conductivity jump of seven orders of magnitude at a temperature around 670 K. Through experimental studies and density-functional theory calculations we confirmed that the conductivity jump takes place at a critical gallium concentration and is induced by crystallization of stoichiometric Ga2O3. This novel mechanism – an insulator-metal transition driven by a heterogeneous solid state reaction within an amorphous matrix – opens up a new route to achieve metallic behaviour in oxides that are expected to exist only as classic insulators.
Insulator–metal transitions belong to the most fascinating phenomena in condensed-matter physics [1]. The transition can be caused by strong electron-electron interactions (Mott transition) [2] or by structural disorder (Anderson transition) [3]. As shown by Anderson [3] and Mott [4], in any non-crystalline material the lowest states in the conduction band are localized. Only for energies above the mobility edge do states become non-localized or extended. If the Fermi energy is below the mobility edge, the material is an electronic insulator, but if the number of electrons increases and the Fermi energy rises above the mobility edge, the material becomes metallic. Most examples of insulator–metal transitions concern transition metal compounds [4,5] as transition metals change their valence state easily. However, this does not exclude the possibility of inducing an insulator–metal transition in a simple binary oxide of a main group element, even without doping. Instead, large deviations from the ideal stoichiometry, i.e. high defect concentrations, provide a high concentration of electronic defects (self-doping). And if, in addition, the oxide is amorphous, there are two phenomena, strong chemical and strong structural disorder, which could result in an insulator–metal transition. We made thin films of highly non-stoichiometric, amorphous gallium oxide with approximate compositions of GaO1.2 using pulsed laser deposition under reducing conditions and Ga2O3 as starting material. The films are insulating but, on heating, they show a sudden jump in conductivity of around seven orders of magnitude at ~670 K (Figure 1a). As the temperature increases towards the insulator–metal transition, β-Ga2O3 nuclei crystallize, requiring more oxygen than gallium. Hence, the surrounding amorphous region becomes even more non-stoichiometric. The material becomes conducting when the gallium concentration in the amorphous region reaches a critical level. Then, the conducting material consists of nuclei of crystalline and stoichiometric β-Ga2O3 surrounded by amorphous and highly non-stoichiometric gallium oxide (Figure 1b).
One key to the success of this new insulator–metal transition can be attributed to the formation of extremely non-stoichiometric and amorphous GaOx as starting material. To obtain insights into its local structure, X-ray absorption spectroscopy at the Ga K-edge was performed at the beamlines of HASYLAB at DESY in Hamburg (Figure 2). Modelling of the extended X-ray absorption fine structure (EXAFS) with FEFF8 [6] indicates that the local structure around the gallium atoms can be described reasonably well by the same oxygen coordination polyhedra as in crystalline β-Ga2O3, i.e. strongly distorted oxygen octahedra and tetrahedra. However, in contrast to β-Ga2O3, which has equal amounts of gallium atoms with octahedral and tetrahedral oxygen coordination, the amorphous and highly non stoichiometric gallium oxide GaO1.2 contains a significantly higher fraction of gallium atoms with tetrahedral oxygen coordination, Gatet:Gaoct ≈ 57:43. The EXAFS results show that the concept of point defects, which is strictly speaking only valid for crystalline structures, should be applicable to amorphous gallium oxide as well. In this sense, the gallium excess of GaO1.2 can be due to gallium interstitials and/or oxygen vacancies.
Figure 2: The local structure of amorphous and non-stoichiometric gallium oxide films, GaO1.2, was investigated by X-ray absorption spectroscopy at the Ga K-edge. (a) EXAFS, k2⋅χ(k), of an as-prepared, amorphous gallium oxide film. (b) Modified radial distribution function G’(R) (Fourier transform of the EXAFS in (a)). The experimental data (black lines) were fitted with a linear combination of theoretical spectra for gallium atoms with tetrahedral oxygen surrounding, Gatet, and octahedral surrounding, Gaoct. The fit (blue lines) yields a distribution Gatet: Gaoct = (57±3):(43±3), i.e. a significantly higher occupation of tetrahedral sites compared to β-Ga2O3 having identical occupation numbers.
Theoretical calculations of the electronic structure of highly non-stoichiometric gallium oxide by means of density functional theory DFT [7] show, however, that the amorphous phase may be considered more appropriately as containing gallium interstitials (donor states close to the conduction band) rather than oxygen vacancies (donor states close to the valence band). By increasing the Ga-excess the number of states in the original optical band gap increases and the energy gap between the highest occupied states and the lowest conduction states decreases (Figure 1c) until the gap is closed at a critical gallium excess – resulting in the observed insulator-metal transition. The results of these investigations have been published in the May issue of Nature Materials. In this context the question arises whether this new mechanism for an insulator–metal transition may have an immediate impact on applications such as electronic devices and permanent data storage. For the latter, the high stability of the material is a key requirement. One drawback at present, however, is that the transition from a metastable to a stable state is only one-way. If a reversible transition was achieved, this would surely have great implications for applications such as multiple read/write storage devices. If the principle is applied to other main group oxide systems, it may be possible to tune the thermal stability of the crystalline regions and realize reversible transitions.
References |
||||||||||||||
|
||||||||||||||
Contact information |
||||||||||||||
Manfred Martin |
||||||||||||||
Further Information |