Virtual 360 Degree tour of the DESY light sources and site (FLASH, PETRA III)
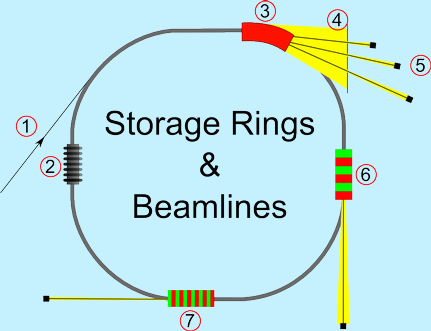
- Injection
- RF system
- Storage Ring/Bending Magnets
- Beamlines
- Experiments
- Wigglers
- Undulators
1. Injection
Prior to entering the actual storage ring, the electrons need to be pre-accelerated to their final energy and speed (in the actual storage ring their energy is only maintained). In the case of PETRA III (and before of the former storage ring DORIS III), this is done in several steps.
Linac II
PIA
DESY II
The first step is the acceleration in Linac II:
Free electrons are generated in long pulses (several microseconds) at the cathode, which is a heated block of negatively charged metal. From this a shorter pulse (ca. 20 nanoseconds) is selected by application of an electrostatic field in the chopper and the beam then narrowed down by a collimator. All this time they get accelerated towards the anode, which is a positively charged ring of metal, whose field guides the electron beam through it and towards the prebuncher.
This whole setup is called the “electron gun”. In the prebuncher the electron beam gets subdivided into bunches ca. 0.3 nanoseconds long. They then reach the actual linac (linear accelerator), where the electron bunches get accelerated.
This is done by synchronizing their movement with the radiation so that they always experience a field with the correct orientation to enhance their energy (represented by the positive part of the wave on the graph). The radiation generated by a “klystron” has the same frequency at which the electron bunches are emitted from the prebuncher (~3 GHz). Basically, the higher up on the wave crest an electron is, the greater the transferred energy and thus acceleration. In the picture the right peak represents the left one a split second later. The trick to maintaining compact particle bunches is to design the RF system so that the center of mass of the bunches (marked by the dotted line) lies a bit away from the peak, although one would get the greatest acceleration there.
If a particle has energy slightly above the average, it gets slightly behind, since it gets less strongly focused in the focusing magnets (quadrupoles) along the linac and has to travel a longer distance through them. It would then experience a lower field and it's energy would drift back towards the mean. Equivalently, particles with too low energy get ahead and experience greater energy transfer. This means particles oscillate around the bunch’s centre of mass and the mean energy if they are within a certain range around it, which leads to a certain bunch size as first generated in the prebuncher using similar principles. After leaving the linac, the electrons already travel close to the speed of light.
For PETRA III (before for the former storage ring DORIS III), two “booster synchrotrons” are used to bring the electrons to their final energy: First PIA and then DESY II. In these, every time the electrons go around, they get an additional boost from an “RF cavity”, which basically works with the same principle as the linac. After leaving DESY II, the electrons have energy of 4.5 GeV and a speed of 99.9999993% of the speed of light.
2. RF system
The RF or radio frequency system is responsible for supplying energy to the electrons as the move around the storage ring. This is necessary since the synchrotron radiation generated for the experiments constantly drains the electrons’ energy. All energy is supplied as electromagnetic radiation with a wavelength of about 0.1 metres (which equates to a freuquency of 3 GHz as before), i.e. as microwaves.
The RF system is made up of three components:
- Klystron
- Waveguides
- RF cavities
A klystron is a powerful microwave amplifier, i.e it takes a weak input signal and generates a strong output signal, which can then be used as energy in microwave form, which in this case is transferred to the electrons. The principle has been known since the discovery of radar technology in the Second World War. Waveguides are coupled to the klystron as a means of transporting the radiation towards the cavity.
- An electron gun as described for the linac provides an intense electron beam.
- A low energy microwave signal intersecting the electron beam divides it into bunches, i.e. generates a pulsed beam. The underlying principle is similar to the linac’s buncher. The electrons are sped up when the intersecting beam is close to the crest and slowed down when it is near its trough. The generated bunches have the same frequency as the intersecting signal, namely 3 GHz.
- When the modulated electron beam passes through the tuned waveguide, a high energy microwave signal is generated. The waveguide is precisely engineered to resonate at the exact needed frequency, similar to a bell resonating at a certain pitch.
- The microwaves then pass down the waveguide to the linac or an RF cavity.
A waveguide in the general sense is a means of efficiently transporting electromagnetic radiation, for example a coaxial cable for the TV signal or an optical fibre.
In the example of the RF system, the waveguide consists of a hollow boxlike metal-structure approx. the size of the radiation’s wavelength.
The radiation then reaches the RF cavity, which is shaped to resonate at 3 GHz as well. Energy is transferred to the passing electrons as described for the linac. This makes up for the energy lost as synchrotron radiation during each cycle.
The whole process has to be timed extremely accurately, since the wave-package traversing the cavity every 200 nanoseconds needs to coincide precisely with a specific phase of the electromagnetic radiation to provide the necessary energy.
3. Storage ring/bending magnets
After leaving DESY II, the electron bunches then enter the storage ring PETRA III (before the former storage ring DORIS III), where all the actual research happens. To allow the electrons to circle the ring for many hours, they travel in a metal tube in which an ultra-high vacuum is maintained, the vacuum chamber. The air pressure in here is only about a hundred billion-th (!) of the outside pressure.
The electrons are kept on their circular path with the help of strong magnetic fields (1.3 Tesla) applied by the bending magnets.
To keep the electrons confined within the small vacuum chamber, elaborate electronics constantly refocus the beam.
Also, the bending magnets are one of the sources of synchrotron light, where a narrow searchlight-like cone of radiation is emitted as the electrons pass the bend.
Other sources are wigglers and undulators.
4. Beamlines
After the beam of light is generated in the storage ring it travels though a system of evacuated steel pipes toward the experiments. The beam line is usually made up of these elements which are used for beam "conditioning":
- A monochromator can filter out a single desired wavelength of radiation with a narrow bandwidth.
The type used for x-rays is a crystal monochromator.The incident “white” x-ray beam (black line) hits a perfect silicon one-crystal at a precisely defined angle θ. The process which is then exploited is called Bragg diffraction. Part of the radiation is diffracted (more or less reflected) off the top layer of atoms, while another part is diffracted off the second layer, a distance d away. These two rays then interfere constructively or destructively depending on their wavelength. The peaks are given by Bragg’s law 2d·sin θ = nλ. The strongest peak and the one that is selected is the one with n = 1. After being diffracted off the two crystals, the beam (red line) faces in the original direction again and a narrow bandwidth around the wavelength λ has been selected .
For radiation in the UV region of the spectrum, a grating monochromator is used. The incident beam is focused onto a grating by the first mirror. This then diffracts the beam, distributing the different wavelengths over a cone of radiation. From this, the desired wavelength can be chosen and the resulting monochromatic beam is focused onto the exit of the monochromator by the second mirror.
- Windows - thin sheets of metal, often beryllium, which transmit almost all of the beam, but protect the vacuum within the storage ring from contamination
- Slits - which control the physical width of the beam and its angular spread
- Focusing elements - one or more mirrors, which may be flat, bent-flat, or toroidal. Lenses are also used. Both helps to collimate (focus) the beam
- Spacing tubes – vacuum tubes which provide the proper space between optical elements, and shield any scattered radiation.
5. Experiments
After travelling through the beam line, the radiation can finally be used in the various experiments conducted at a light source. Simply put it is made up of three parts:
- Sample stage - for mounting and manipulating the sample under study and subjecting it to various external conditions, such a varying temperature, pressure etc.
- Radiation detectors for measuring the radiation which has interacted with the sample as well as detectors for other products
- Computers to collect and analyze the data coming from the detectors
For a full overview of the techniques employed by researchers in the study of matter view the “Experimental Techniques” primer.
6. Wigglers
These so called “insertion devices” are a more effective way of generating synchrotron radiation than bending magnets. In these, the electrons are forced on a slalom course by an array of permanent magnets. Wigglers provide radiation with the same properties as bending magnets, only with an intensity enhanced by the number of magnet poles.
7. Undulators
These "insertion devices" use weaker magnetic fields and shorter magnets. The electrons move in weak undulations of short period and the radiation cones generated in each period overrlap in a way that they interfere and give the radiation special properties.
A full explanation of the mechanisms behind the generation of synchrotron radiation is found in the “SR” primer.